Introduction to Theoretical Yield:
Understanding the theoretical yield of a chemical reaction is vital for scientists and chemists to maximize efficiency and optimize their processes. Theoretical yield refers to the maximum amount of product that can be obtained from a given quantity of reactants, assuming ideal conditions and complete conversion. In this blog post, we will explore the importance of calculating theoretical yield, the factors influencing it, and provide illustrative examples to help you grasp this concept effectively.
The Importance of Calculating Theoretical Yield:
1. Resource Optimization:
By calculating theoretical yield, scientists can determine the appropriate amounts of reactants required to maximize yield while minimizing waste. This information helps streamline the production process and minimize resource consumption.
2. Quality Control:
Theoretical yield calculations aid in ensuring the quality and purity of the final product. By knowing the maximum amount of product that should be obtained, it becomes easier to compare the actual yield with the expected yield, allowing for adjustments in reaction conditions if necessary.
3. Predictability and Safety:
Theoretical yield calculations provide scientists with a clear understanding of what to expect from a reaction, helping them identify potential hazards or unexpected outcomes. This knowledge enables them to implement measures to handle any challenges or deviations.
Factors Influencing Theoretical Yield:
1. Stoichiometric Ratios:
The stoichiometric ratios between reactants and products, as determined by the balanced chemical equation, play a crucial role in calculating theoretical yield. The stoichiometry ensures the correct proportional relationship between reactants and products in a balanced equation.
2. Limiting and Excess Reactants:
The theoretical yield is also affected by the presence of limiting and excess reactants. The limiting reactant is the reactant that is completely consumed, determining the maximum amount of product that can be obtained. Excess reactants, on the other hand, cannot be entirely converted and do not contribute to the theoretical yield.
3. Reaction Efficiency:
The efficiency of a reaction is a significant factor influencing the theoretical yield. Factors such as temperature, pressure, catalyst usage, and reaction environment can impact the overall efficiency, ultimately affecting the reaction's theoretical yield.
Example Calculations:
Let's consider a simple example to demonstrate one way to calculate theoretical yield.
Example 1: Hydrogen and Oxygen Reaction
Balanced Reaction: 2 H₂ + O₂ → 2 H₂O
(Phases of reactants/product omitted for clarity)
Let’s consider a scenario where we react 4 moles of hydrogen (H₂) and 2 moles of oxygen (O₂):
Step 1: Convert the given moles of each reagent into the moles of the desired product (water, H₂O), using the appropriate stoichiometric ratios from the balanced equation.
4 moles of H₂ × (2 moles of H₂O / 2 moles of H₂) = 4 moles of H₂O
2 moles of O₂ × (2 moles of H₂O / 1 mole of O₂) = 4 moles of H₂O
Step 2: Determine the limiting reactant by comparing the calculated moles of product from each reactant.
In this case, both reactants produce 4 moles of H₂O. Thus, neither reactant is limiting.
Step 3: Theoretical Yield
Since there are no limiting reactants in this example, the theoretical yield will be 4 moles of water (H₂O).
Example 2: Calculating the Theoretical Yield for an Elimination Reaction (A more representative example of a real reaction that we may encounter in organic chemistry!)
What is the theoretical yield for the elimination reaction of 25.0 g of stilbene dibromide (SDB) with 20.0 g KOH to produce diphenylacetylene (DPA)?

As we can see, the chemical reaction is provided. We can use the line-angle structures along with a periodic table to calculate the molar masses of the reagents and product (here the solvent is ethylene glycol).
Molar mass (KOH): 56.1056 g/mol
Molar mass (Stilbene Dibromide): 340.058 g/mol
Molar mass (Diphenylacetylene): 178.24 g/mol
The reaction is unbalanced. However, if we know that the stoichiometric amount of KOH:SDB is a 2:1 mole ratio, we can begin the process for determining the limiting reactant which we will need in order to calculate the theoretical yield. Also, for every mole of the stilbene dibromide that we introduce into the reaction, we will produce one mole of DPA (a 1:1 mole ratio).
Steps 1, 2, and 3: Convert the given grams of each reagent to moles of each reagent using molar masses, and then use the mole ratio to convert into the moles of the desired product. Lastly, use the molar mass of the desired product to convert moles of product formed from each reagent into units of grams!
We combined these three steps into one linear process just like you will see in any general chemistry stoichiometry curriculum, but you can also separate them out into individual steps if you needed to do so (e.g. You may want to observe the ratio of reagents, so you could stop after the first bracket to compare the moles of the two reagents, we see about a 5:1 KOH:SDB ratio in this example!).


Now we can easily see which reagent was used up first (or produced less product). This will be our limiting reactant!
Step 4: Compare the grams of product formed from each reagent and select the limiting reactant.
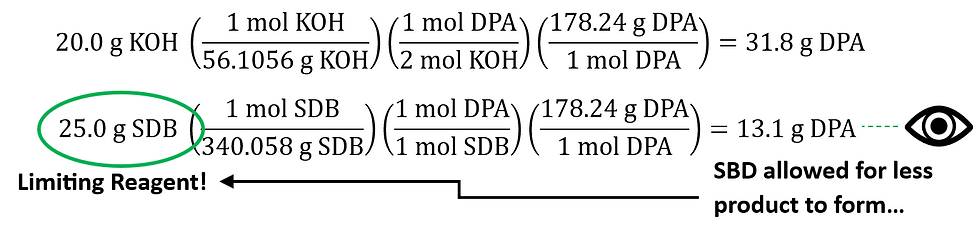
Step 5: Determine the theoretical yield of the desired product.
By completing steps 1-4 in linear fashion, we have also determined the theoretical yield in grams for PDA! The maximum amount of PDA that is theoretically possible could be determined in moles or grams depending on what you need. You would just stop after step 2 above to obtain the theoretical yield in moles instead of grams. However, we can measure masses in the lab using a balance, so most likely you will want to convert to grams of product instead of moles. This will set you up to easily calculate a percent yield for your reaction after performing the synthesis in the lab. We will discuss this process below.

As with many concepts in chemistry, there are more than one valid route for determining the limiting reactant (e.g. ratios of reagents), but we find that the method described above is more straightforward and can allow for the simultaneous determination of the limiting reactant and theoretical yield in grams.
Conclusion:
Understanding and calculating theoretical yield is crucial for scientists and chemists to optimize their processes, reduce resource wastage, and ensure product quality. By considering the stoichiometric ratios, limiting reactants, and reaction efficiency, you can accurately determine the theoretical yield of a chemical reaction. By doing so, we unveil the potential hidden within chemical reactions, bringing us one step closer to achieving greater scientific advancements.
Introduction to Percent Yield:
In the realm of chemistry, determining the efficiency of a chemical reaction is crucial for researchers, scientists, and students alike. One key parameter used to evaluate this efficiency is the percent yield. In this blog post, we will explore the concept of percent yield, learn how to calculate it, and provide specific examples to illustrate its application in real-world scenarios.
Understanding Percent Yield:
Percent yield is a measurement that determines how much product is obtained in relation to the maximum possible yield. The actual yield (the amount of product obtained in a reaction) is compared to the theoretical yield (the maximum amount of product that could be obtained under perfect conditions). A high percent yield indicates a more efficient reaction.
Calculating Percent Yield:
To calculate percent yield, follow this simple formula:
Percent Yield = (Actual Yield / Theoretical Yield) x 100 %
Theoretical yield is typically obtained using stoichiometry, where the balanced equation and molar masses of reactants and products are utilized. The actual yield is determined experimentally by conducting the reaction and measuring the final product.
Examples:
Let's delve into a few specific examples showcasing how percent yield calculations work.
Example 1: Synthesis of Ammonia
Suppose we want to synthesize ammonia (NH3) by reacting nitrogen gas (N₂) with hydrogen gas (H₂).
Balanced Reaction: N2 + 3 H₂ → 2 NH3
(Phases of reactants/product omitted for clarity)
If we performed the reaction and obtained 10 grams of ammonia, and the theoretical yield, calculated using stoichiometry, was 12 grams, we can calculate the percent yield as follows:
Percent Yield = (10 g / 12 g) x 100
Percent Yield = 83.3 %
Example 2: Calculating the Percent Yield for an Elimination Reaction (A more representative example of a real reaction that we may encounter in organic chemistry!)
Let’s assume that we ran the following reaction in the laboratory and obtained an actual yield (the mass of the product that we isolated after the reaction) of 12.2 g of the product (DPA). What is the percent yield?

Recall that we have already determined the theoretical yield in previous example. So now we just need to plug into the formula for percent yield and compute.

From the percent yield, we can conclude proceeded successfully with high yield. The lab experiment requires only minor optimizations (if any!).
Often, in organic chemistry, more than one reaction is required to reach the desired product. Thus, we will need to calculate an overall percent yield for a multi-step synthesis. Take the example set of 2 reactions below:
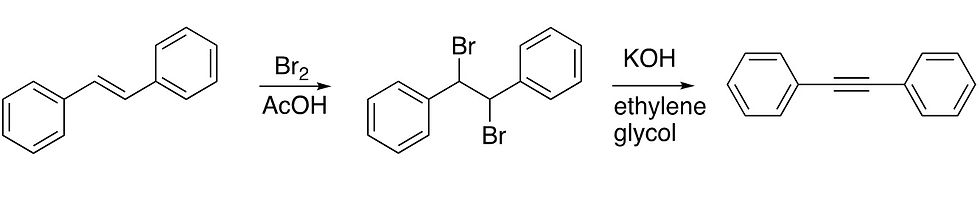
Molar Mass (Bromine): 159.81 g/mol
Molar Mass (Stilbene): 180.25 g/mol
Molar mass (KOH): 56.1056 g/mol
Molar mass (Stilbene Dibromide): 340.058 g/mol
Molar mass (Diphenylacetylene): 178.24 g/mol
In this example, we can see that we have already calculated the percent yield going from the bromide to the alkyne, but we must now also consider the first step, going from the alkene to the dibromide in the solvent acetic acid (AcOH). For the reaction in step 1, let’s say we started with 31.5 grams of stilbene (SB) and 41.9 g of bromine and that the bromine has been added in excess. Theoretically, this should yield 59.5 g of SDB (based on the limiting reagent!).
The reaction actually afforded 25.0 g of SDB (the actual yield, obtained from running the reaction and isolating the product in the lab) corresponding to a 42.1 % yield in step 1. Now to calculate the overall yield of the entire reaction (both steps!), we can use the following pathway:
Step 1: Convert percent yields from each step to decimal fractions.

Step 2: Multiply the decimal fraction yields for each step, and convert back to a percentage.

Conclusion:
Percent yield calculations provide a valuable measure of reaction efficiency. By comparing the actual yield to the theoretical yield, chemists can assess how well a reaction is performing and identify areas for improvement or optimization. It is crucial to note that a percent yield of 100% is rarely achieved due to various factors such as impurities, side reactions, and experimental limitations. Calculating percent yield helps researchers refine their techniques and obtain a clearer understanding of the reaction's limitations.
By mastering the concept of percent yield calculations, chemists can enhance their experimental approach, make informed decisions, and work towards improving the overall efficiency of chemical reactions.
Commenti